Ironmaking and steelmaking activities are excellent examples of chemical processes taken beyond the textbooks and laboratory and implemented in industry on a grand scale. The New Zealand Steel plant at Glenbrook is unusual in that the huge blast furnaces that dominate the processes at most steel works around the world are not present. This has eventuated because of the nature of the ore mined locally and used in the process. Early pioneering experiments failed to successfully smelt iron from New Zealand's west coast ironsand deposits because the magnetite ore (Fe3O4), as it is known, is locked in with minerals such as titania and silica. These bonded oxide impurities are collectively known as "gangue".
The success of this direct reduction process of ironsands on a commercial scale was a hard-fought technological battle, culminating in a world first achievement for New Zealand Steel.
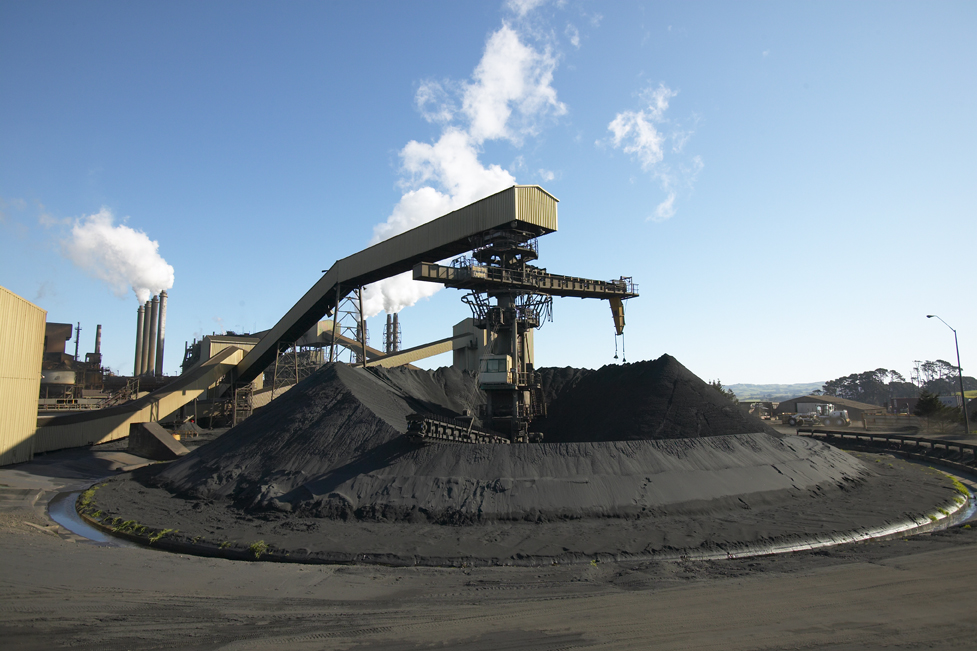
At the Waikato North Head mine, the ironsand is concentrated by magnetic and gravity separation processes to increase the iron content and decrease the amount of loose impurities. This product, which is known as primary concentrate or PC, is pumped in a slurry to the steel mill where it is dewatered and stockpiled.
At the mill, to prepare the materials for chemical reduction, coal and primary concentrate (ironsand), along with a small amount of limestone (CaCO3), are mixed together before being fed into the top of each of the four multi-hearth furnaces.
The intense heat generated in these furnaces dries the feed material and drives off the coal volatiles comprising hydrocarbons such as tars, oils propane, butane and methane. Combusting a fraction of these volatiles provides the energy needed for this process. The excess volatiles are burnt in an afterburner and the energy is utilised for generating electricity. Rabble arms, supported on a central rotating vertical shaft, rake the material from hearth to hearth as it drops down the 12 hearth layers of each of the four furnaces. Materials exiting the multi-hearth process have been dried and heated to about 650°C and the coal has been converted to char through the liberation of volatiles. It is now suitable for feeding into the kilns.
The four horizontal rotary kilns are the heart of the reduction process. The residual char (carbon) remaining after the coal was heated is used to achieve the chemical reduction of iron oxide to iron.
A number of chemical reactions occur in the kilns because coal and ironsand are not pure substances like laboratory chemicals. Heat for the reactions is provided by the combustion of carbon in air, which is introduced through burners along the length of the rotating kiln. The main reactions are:
C | + | O2 | → | CO2 | + | Heat |
CO2 | + | C | → | 2CO | ||
Fe3O4 | + | CO | → | 3 FeO | + | CO2 |
FeO |
+ | CO | → | Fe | + | CO2 |
Fe3O4 | + | 4C | → | 3Fe | + | 4CO |
As long as temperatures are high enough and excess carbon is available, the CO2 is reduced to CO (second equation above), which can once again participate in the reduction of iron oxide.
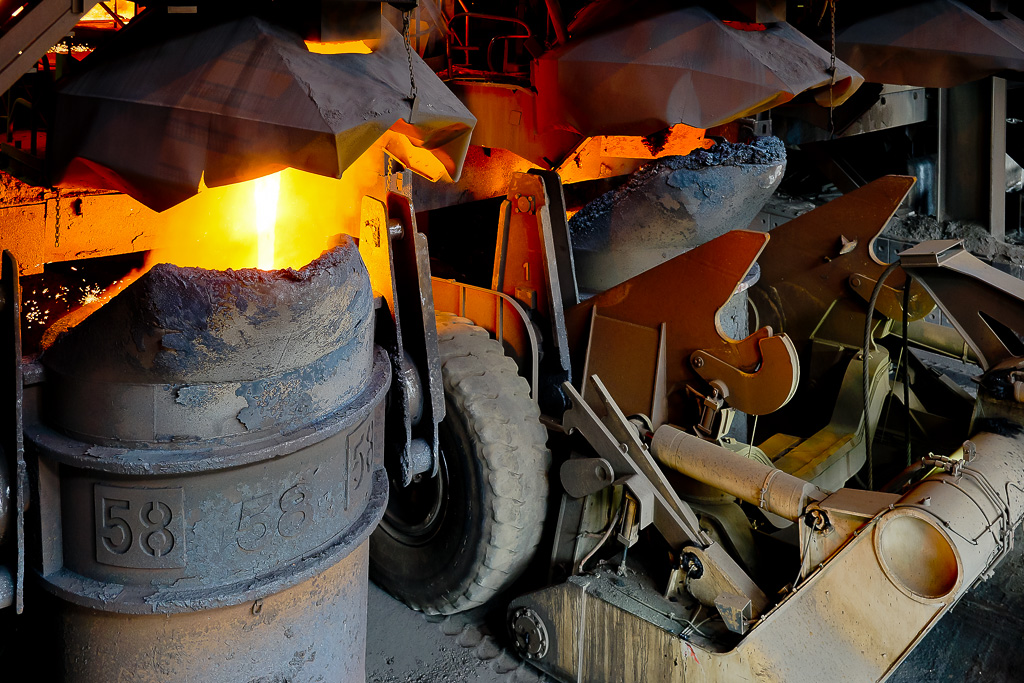
The char is burnt at a predetermined rate by the air blown in through the fans mounted on the kiln shell. The hot gases generated during combustion raise the temperature of the kiln refractory lining (bricks) and as the kiln rotates (at one revolution every two minutes), the bed of iron sand, char and limestone slides over hot refractory. This elevates the product temperature still further to around 900°C at the exit. This process allows the carbon monoxide molecules to strip the oxygen away from the iron oxide.
The limestone added to the coal stockpile is "burnt" in the kiln.
CaCO3 | + | Heat | → | CaO | + | CO2 |
Kiln waste gas has a high temperature upon exiting the kiln and consists of combustible components mainly CO. As in the case of the multi-hearth furnace waste gas, kiln off gas is also passed through an afterburner and heat exchanger to generate high pressure steam, which drives a turbine/generator set for electricity production.
The quality of the kiln product, (reduced primary concentrate and char also known as RPCC), in terms of the percentages of metallic iron and carbon, is most stable when material input and operating conditions are kept constant. Hence, much effort is made to minimize changes in ore and coal composition. The kilns also operate continuously on a planned campaign of 24 months between maintenance shutdowns to keep conditions as near to constant as possible.
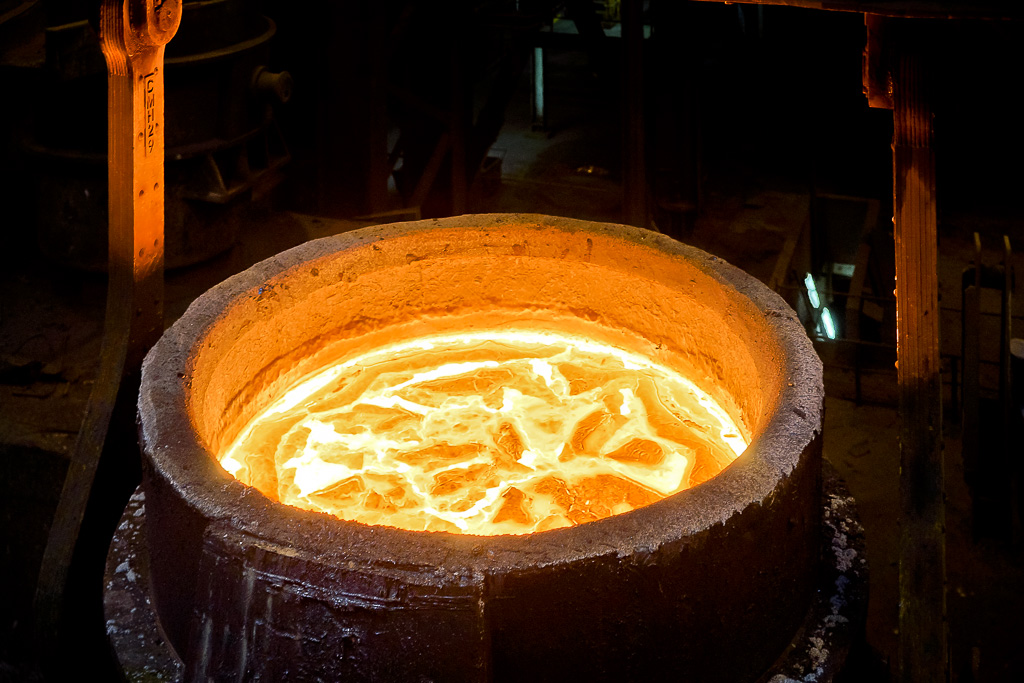
In each of the two melters, electricity is used to achieve the final reduction of iron oxides present in the RPCC and to liquify the iron. For the reduction, the carried over carbon (char) is used and it is very important that a constant amount is present in RPCC in order to produce quality iron in the melter. The mix of iron and oxide impurities is heated above melting temperature so that the iron and slag may be tapped as liquids from the furnace at around 1500°C. The molten iron has a dissolved carbon content of approximately 3.6% as well as some silicon, manganese, vanadium, and titanium. Slag, which floats on top of the iron, has the function of removing impurities from the iron and its chemical composition and temperature to a large extent determine the chemistry and hence quality of the iron.
The CaO produced in the kiln from the limestone added with the coal is used as a flux to remove sulfur and other impurities from the molten iron in the melter.
CaO | + | FeS | + | C | → | CaS | Fe | CO |
The slag is made up of a range of oxides and its formation is essential to remove undesirable elements such as silicon, titanium and sulfur. Phosphorous, another undesirable element in steelmaking, is not removed from the iron and it is therefore very important to minimize its presence in the feed materials right at the outset.
An important slag parameter is the so-called basicity index. This is the ratio of the basic slag components such as CaO, MgO and FeO to the acidic slag components such as SiO2, TiO2, and Al2O3. The relative amounts of these oxides determine, to a large extent, the physical properties of the molten slag such as melting temperature, viscosity and fluidity which in turn determine its ability to remove impurities from the iron.
Inevitable by-products of ironmaking are hot gases emitted in large volumes from the multi-hearth furnaces, kilns and melters. These hot gases have a considerable energy value so are burned as a fuel in after-burners and are used to make high-pressure steam for generating electricity.
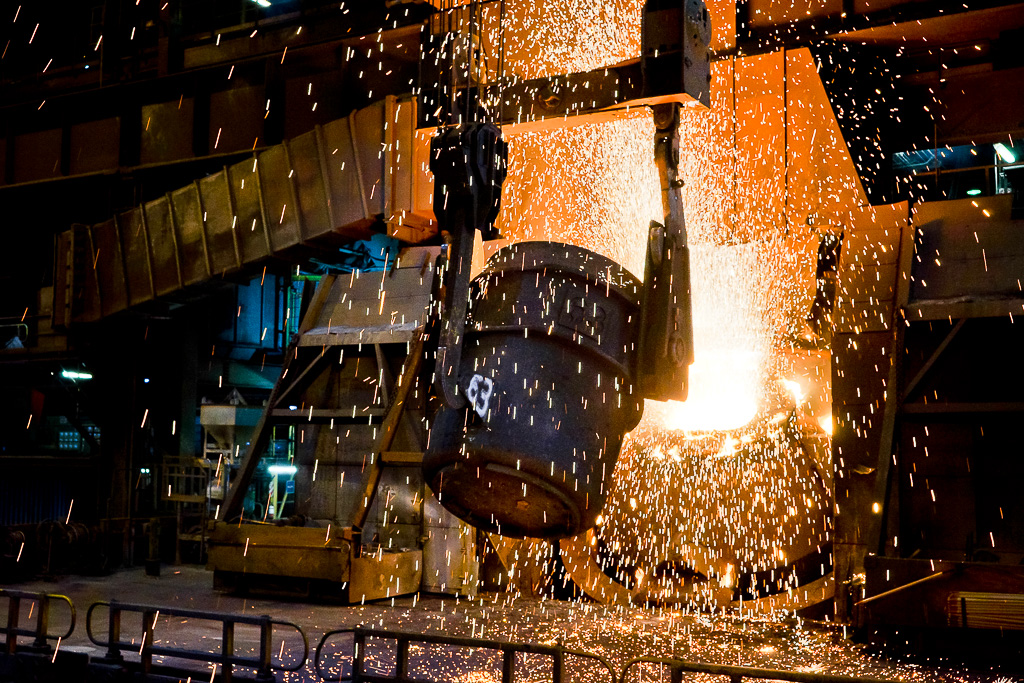
The final product from the melter process is liquid iron, which is tapped every four hours into refractory lined steel containers called ladles and transported to the steel plant where it is converted and refined into liquid steel.
The typical hot metal chemistry of the molten pig iron transferred to the steelmaking process is:
Iron (Fe) | 95.50% |
Carbon (C) | 03.40% |
Vanadium (V) | 00.42% |
Titanium (Ti) | 00.16% |
Silicon (Si) | 00.11% |
Manganese (Mn) | 00.33% |
Sulfur (S) | 00.06% |
Phosphorus (P) | 00.06% |